板材單點(diǎn)增量成形夾具設(shè)計(jì)【含4張CAD圖】
【需要咨詢購(gòu)買全套設(shè)計(jì)請(qǐng)加QQ1459919609】圖紙預(yù)覽詳情如下:
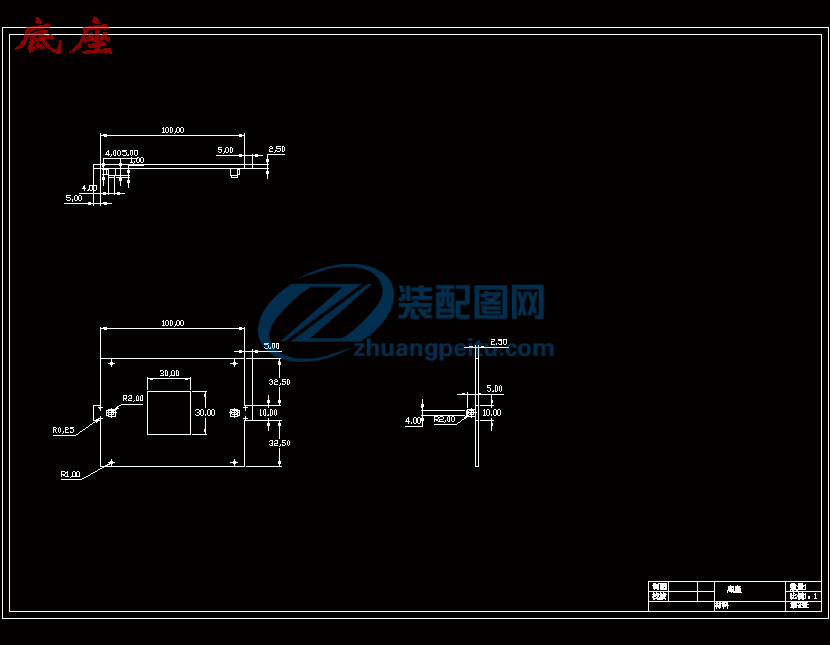
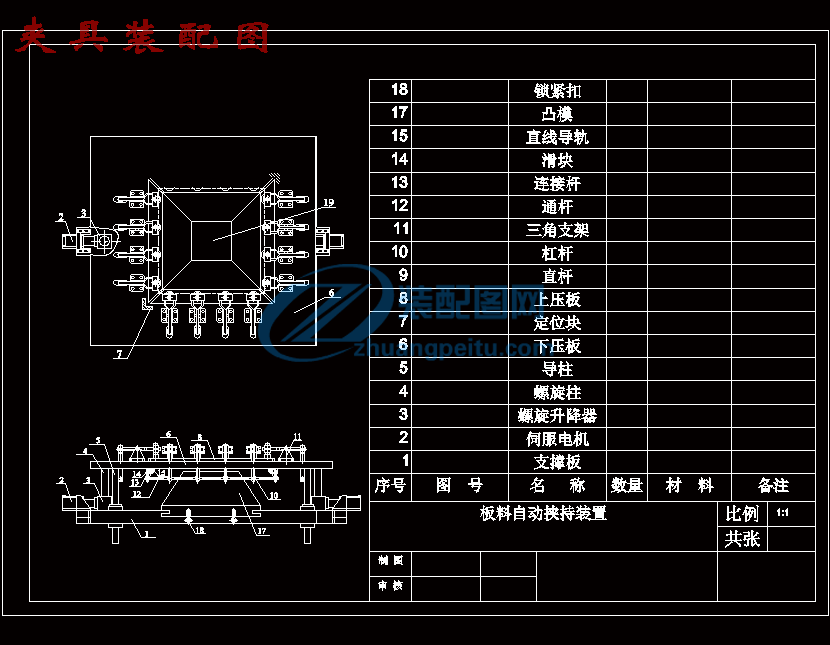
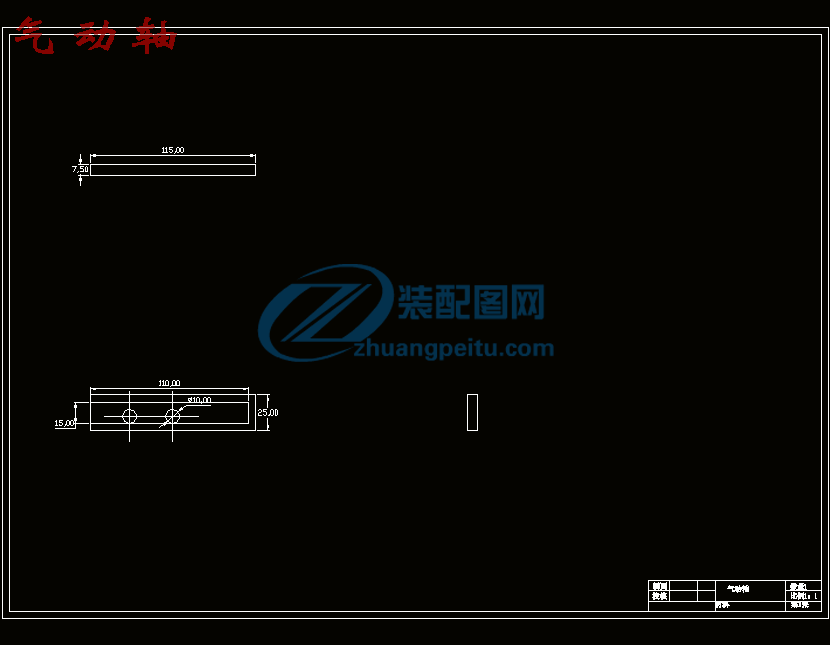

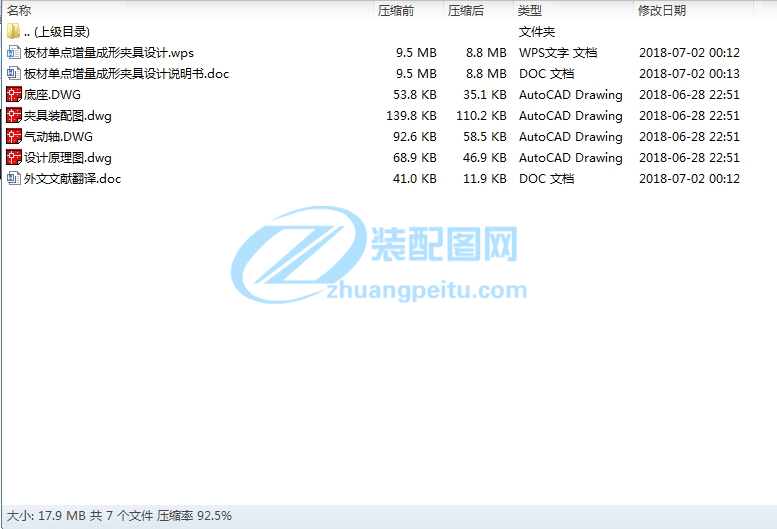
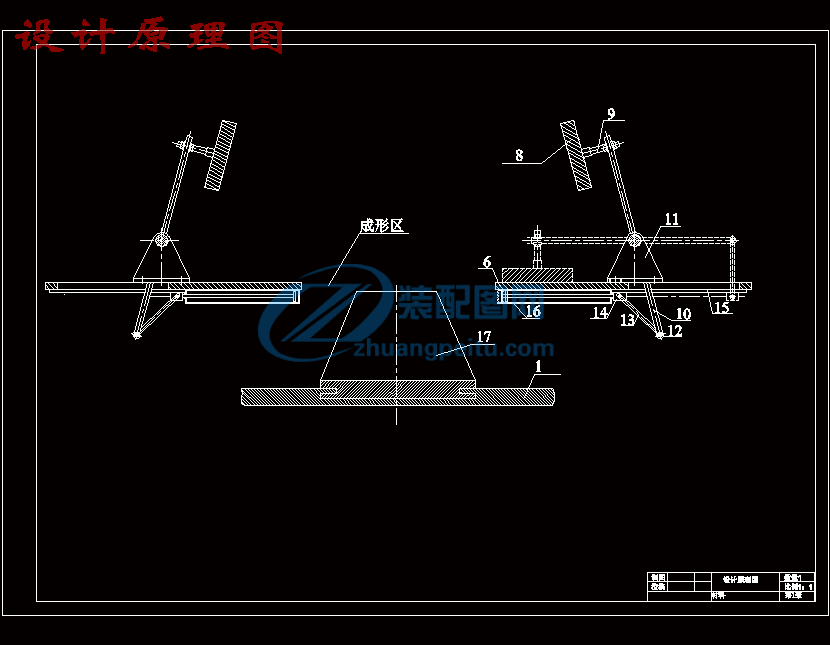

附錄Ⅰ外文文獻(xiàn)及翻譯外文文獻(xiàn)Formability in single point incremental forming: A comparative analysis of the state of the artSingle point incremental forming (SPIF) is a method of manufacturing components from sheets of material, with the advantage of little to no customised tooling and otherwise generic setup [1]. This makes it ideal for producing sheet metal prototype components before investing in a stamping mould, or for one-off customised components.SPIF is a type of incremental sheet forming (ISF), a class of processes which includes spinning and shear forming [2]. SPIF has the advantage over a method such as spinning of being able to form asymmetric shapes. In the 2005 paper, Jeswiet et al. [3] succeeded in bringing all the current knowledge at that time and synthesising it into a comprehensive review of the progress and state of the art of asymmetric ISF processes. The decade of research since then calls for an updated review of the progress and understanding of SPIF, including incremental forming of polymer sheets ?rst explored in 2008 [4].This paper comprises a review of literature on single point incremental forming, speci?cally to present the process param- eters that in?uence the formability of the material during forming. Organising the results of this investigation will assist in creating straightforward parameter guidelines and instructions useful for future research and manufacturing real components with SPIF.While commercial and industrial SPIF components have been made in the past, they can be so complex that ‘trial and error’ becomes the most feasible development technique, as using FEA (?nite element analysis) would be too computationally expensive. Therefore, a signi?cant challenge is how to develop it into an industrial process using methods more sophisticated than trial and error.The aim of this literature review is to collect relevant data from experimental papers and draw conclusions on maximising the formability of the material used in SPIF. The other aspects of SPIF that are not systematically covered in this review are forming forces, surface quality, geometric accuracy, and resultant material properties.Formability is most commonly quanti?ed by ?nding the maximum wall angle (Fmax) to which the material can be formed before failure occurs [5], with respect to the horizontal plane.Typically a simple shape, such as a cone or pyramid, is used to determine this maximum wall angle. Multiple parts can be formed, each one with a steeper wall angle than the previous, until a part breaks [6].Another option is a shape where the wall angle changes from shallow to steep, for example the variable wall angle conical frustum (VWACF) reported in 2007 by Hussain et al. [7]. If this part is used, only one test is needed to determine the wall angle where failure occurs. In the same paper, Hussain et al. [7] compared the VWACF results and results from straight-wall tests and found the latter overestimated Fmax by less than 4% due to its higherstiffness. Therefore, if the exact wall angle at fracture is required for straight wall parts, the VWACF results should be further tested with conical or pyramidal frustums. Any formability test, however, if it is repeated accurately, should give consistent results such that the general effect of a process parameter can be determined. The general effect, further explained in ‘‘Results and discussion’’ section, provides the data that is analysed in this literature review.Many different materials have been used in SPIF, including a variety of metals [3], polymer sheets [4], and other sheet materials such as sandwich panels [8], with a wide range of formability among them. This review will not examine formability limits of speci?c materials but will instead list them in the parameter analyses to allow comparison between experiments with the same material, for example PVC or AA3003-O. Material type can be seen as the base upon which all other parameters are selected.The thickness of the undeformed material blank is an important parameter and has signi?cant effects on the SPIF process and ?nal part, especially the force needed to deform the sheet which increases with increasing thickness [9]. Sheet thickness is also a factor in the sine law equation for shear forming, where the ?nalthickness (tf) of a part can be calculated from the initial thickness (ti) and the wall angle from horizontal (F). The equation is tf = ti * sin(90 — F) and has been shown to be accurate for SPIF parts formed in a single pass [10].The absolute values of thickness are not important in this review, as unique material properties mean a 2 mm sheet of one material performs differently to a 2 mm sheet of a different material [3]. Only the general effect of increasing or decreasing the thickness of the undeformed blank has been studied.Tool parameters The tool or punch used in SPIF to deform the sheet has traditionally been one of two types. Firstly, a solid hemispherical tool [2], and secondly, a tool with a ball bearing in a socket, allowing it to roll freely over the sheet [6]. As progress in SPIF and incremental forming in general developed, the types of tools expanded to include ?at-ended and other shaped tools [11]. Fig. 1 shows the dimensions used to describe 3 main types of tools. Theadvantages of one type of tool over another have been a consistent area of research for some time, for example Kim and Park [12] and more recently Cawley et al. [13]. Tool diameter and tool type are two parameters studied in this literature review.The size and end-shape of the tool in?uence the mechanisms of the forming process. The size and shape of the area of contact between the tool and sheet can affect process aspects such as generated friction [14], observed forces [15], and pressure [16].The tools can be made from different materials, and the interaction between the tool material, blank material and lubrica- tion in?uence the friction conditions seen during the process [17]. Currently there have been no published journal papers examining the effect of different tool materials on formability in SPIF, therefore it is not able to be included in this literature review.Toolpath parameters The toolpath used in SPIF can be the equivalent of a machining operation such as Z-level ?nishing, though the tool does not cut the material. The motion of the tool is de?ned by the same parameters used in machining operations. Feed rate is the velocity of the tool as it moves over the sheet, typically de?ned in mm/min. Step down is how far the tool presses into the sheet with each circuit. Spindle speed is how fast the tool spins, speci?ed in rotations per minute (rpm).Toolpath parameters in?uence the generated friction by the movement and rotation of the tool [18], and the feed rate and step down de?ne the deformation rate of the material.The relative rotation directions of the tool and toolpath determine the ‘milling mode’; either conventional or climb milling, to use the standard machining terms. If the tool and the toolpath are both moving clockwise or counterclockwise, it is conventional milling, and if they are rotating in different directions, it is climb milling. Climb milling is the most commonly used mode in SPIF, as the friction is reduced by the tool effectively ‘rolling’ over the sheet as it forms [19]. While cutting tools typically rotate in a clockwise direction, and therefore specifying toolpath direction might be enough for the reader to assume which milling mode is being used, it is not as thorough as de?ning conventional or climb (‘rolling’) milling as the utilised machining mode.Geometry The shape of the incrementally formed part affects the strains and therefore the formability of the material. The geometry is an aspect which is not covered in this literature review due to the complexity of analysing the many different shapes and dimensions used across the studied papers.In manufacturing a practical component, a draft angle analysis can be performed on the CAD model of the part to highlight which walls are steeper than a speci?ed angle. Using the maximum wall angle for that material in the analysis will show whether there are any sections which may be too steep to form in a single SPIF pass. If there are no sections steeper than the permitted angle, it is likely that the part will succeed [20]. The curvature of the part, from straight walls to tight curves, affects the types of strains (plane, biaxial) developed during the process. For example strains from a conical frustum with 100 mm radius compared to the edge of a pyramidal frustum with 10 mm radius, as presented in Filice et al.Since the process was ?rst developed, research has been conducted into modi?cations of the basic SPIF process. For example, many different methods of heating the workpiece havebeen explored. Du?ou et al. [23] in 2007 used a laser to improve Fmax of TiAl6V4 sheets by more than 208. The same Titanium alloy was heated up to 400 8C with band heaters installed in the blankholder in Palumbo and Brandizzi [24], and an improvement in formability was observed. The use of electric current through the tool and the sheet has been explored in recent years, also applied to TiAl6V4 [25] and other materials such as AA6061-T6 [26] with resulting formability improvements. As this literature review studies process parameters for basic SPIF, parameters relevant to hot SPIF and electric SPIF are not examined.This work Research question What does the literature say about the effects of SPIF process parameters on formability?Hypothesis Each parameter has an optimal operating range which is a function of other parameters. They are interdependent in various combinations.This literature review is undertaken as a ‘systematic quantita- tive literature review’. This type of literature review is de?ned in Pickering and Byrne [27]. The process has been tested by multiple students and researchers, and produces repeatable and high quality results.This technique is highly applicable to the area of SPIF parameters due to the quantitative nature of the data input, and as will be seen in the tabulated results, allows effective comparisons of parameter values between multiple papers. The selected parameters that have been studied in this review are universal to every single pass SPIF process, and more specialised processes such as applying electric current or external heat are not addressed.Many SPIF papers have been published in the area of formability, however the inclusion criteria highlighted below are used to select a high quality collection of papers that are a good representation of the wider ?eld.Details of the process steps as they relate to this literature review are shown below. Formability in single point incremental forming. 中文翻譯單點(diǎn)增量成形過程中成形性的研究現(xiàn)狀單點(diǎn)增量成形(SPIF)是一種用片材制造零件的方法,具有很少或沒有定制工具和其他通用裝置的優(yōu)點(diǎn)[1]。 這使其成為在投資沖壓模具或一次性定制部件之前生產(chǎn)鈑金原型部件的理想選擇。SPIF 是一種增量成型(ISF) ,這是一類包括旋壓和剪切成型的工藝[2]。 SPIF 比諸如能夠形成不對(duì)稱形狀的旋轉(zhuǎn)方法具有優(yōu)勢(shì)。 在 2005 年的論文中,Jeswiet 等人 [3]成功地將當(dāng)時(shí)所有的知識(shí)綜合起來,并將其綜合成為對(duì)不對(duì)稱ISF 過程的進(jìn)展和現(xiàn)狀進(jìn)行綜合評(píng)述。 自那時(shí)起的十年研究要求對(duì) SPIF 的進(jìn)展和理解進(jìn)行更新審查,包括 2008 年首次探索的增量成形聚合物片[4]。本文包括對(duì)單點(diǎn)增量成形的文獻(xiàn)進(jìn)行回顧,特別介紹影響成形過程中材料成形性的工藝參數(shù)。 組織調(diào)查結(jié)果將有助于創(chuàng)建直觀的參數(shù)指南和說明,以便將來用 SPIF 研究和制造真實(shí)組件。雖然商業(yè)和工業(yè) SPIF 組件過去已經(jīng)制造出來,但它們可能非常復(fù)雜,以至于“試錯(cuò)”成為最可行的開發(fā)技術(shù),因?yàn)槭褂?FEA(有限元分析)的計(jì)算過于昂貴。 因此,一個(gè)重大挑戰(zhàn)是如何使用比試驗(yàn)和錯(cuò)誤更復(fù)雜的方法將其發(fā)展成為工業(yè)過程。這篇文獻(xiàn)綜述的目的是收集實(shí)驗(yàn)論文的相關(guān)數(shù)據(jù)并得出最大化 SPIF 材料成形性的結(jié)論。 SPIF 的其他方面未在本文中進(jìn)行系統(tǒng)的介紹,它們正在形成力量,表面質(zhì)量,幾何精度以及由此產(chǎn)生的材料屬性。成形性通常通過找出材料在失效前形成的最大壁角(Fmax)[5] 與水平面之間的關(guān)系進(jìn)行量化。通常使用簡(jiǎn)單的形狀,例如圓錐或金字塔來確定該最大壁角??梢孕纬啥鄠€(gè)部件,每個(gè)部件都具有比先前更陡的壁角,直到部件斷裂[6]。另一種選擇是壁角從淺到陡變化的形狀,例如 Hussain 等人 2007 年報(bào)道的可變壁角圓錐截頭體(VWACF) 。 [7]。如果使用這部分,只需要一個(gè)測(cè)試來確定發(fā)生故障的墻角。在同一篇論文中,Hussain et al。 [7]比較了 VWACF 的結(jié)果和直墻試驗(yàn)的結(jié)果,發(fā)現(xiàn)后者高估了 Fmax 由于其較高的天氣而低于 4%。因此,如果直壁部分需要確切的骨折壁角度,則 VWACF 結(jié)果應(yīng)進(jìn)一步用錐形或錐形平截頭體進(jìn)行測(cè)試。任何可成型性測(cè)試,但是,如果重復(fù)準(zhǔn)確,應(yīng)該給出一致的結(jié)果,以便可以確定過程參數(shù)的一般影響。 “結(jié)果與討論”一節(jié)中進(jìn)一步解釋了總體效應(yīng),該文獻(xiàn)提供了該文獻(xiàn)綜述中分析的數(shù)據(jù)。在 SPIF 中已經(jīng)使用了許多不同的材料,包括各種金屬[3],聚合物片材[4] 和其他片材材料,如夾層板[8] ,其中具有廣泛的可成形性。該評(píng)論不會(huì)檢查特定材料的成形性限制,而是將其列入?yún)?shù)分析中,以便可以在相同材料(例如PVC 或 AA3003-O)之間進(jìn)行實(shí)驗(yàn)對(duì)比。材料類型可以被視為選擇所有其他參數(shù)的基礎(chǔ)。未變形材料坯料的厚度是一個(gè)重要的參數(shù),對(duì) SPIF 工藝和最終部件具有顯著影響,特別是隨著厚度的增加,片材變形所需的力變大[9]。最終,板料厚度也是剪切成型正弦定律公式中的一個(gè)因素可以從初始厚度(ti)和水平角度(F)計(jì)算部件的厚度(tf) 。該方程是 tf = ti * sin( 90 - F) ,并且已經(jīng)證明對(duì)于單次通過形成的 SPIF 零件是準(zhǔn)確的[10]。在這篇綜述中,厚度的絕對(duì)值并不重要,因?yàn)楠?dú)特的材料特性意味著一個(gè)材料的 2 mm 薄片與 2 mm 不同材料的薄片表現(xiàn)不同 [3]。已經(jīng)研究了僅增加或減小未變形空白的厚度的一般效果。刀具參數(shù) SPIF 中用于使紙張變形的工具或沖頭傳統(tǒng)上屬于兩種類型之一。首先是一個(gè)堅(jiān)固的半球形工具[2],其次是一個(gè)帶球軸承的工具,允許它在板上自由滾動(dòng)[6]。隨著 SPIF 和增量成形技術(shù)的發(fā)展,一些工具已經(jīng)擴(kuò)展到包括端部和其他形狀的工具[11]。圖 1 顯示了用于描述 3 種主要類型工具的尺寸。一種工具相對(duì)于另一種工具的優(yōu)點(diǎn)是一段時(shí)間內(nèi)的一致研究領(lǐng)域,例如 Kim 和 Park [12]以及最近的 Cawley 等人。 [13]。刀具直徑和刀具類型是這篇文獻(xiàn)綜述中研究的兩個(gè)參數(shù)。工具的尺寸和端部形狀影響成形過程的機(jī)制。工具和片材之間接觸面積的大小和形狀可能會(huì)影響過程方面,如產(chǎn)生的摩擦力[14],觀察力[15] 和壓力[16]。刀具可以由不同的材料制成,并且刀具材料,坯料和潤(rùn)滑劑之間的相互作用影響過程中所見的摩擦條件[17]。目前還沒有關(guān)于不同工具材料對(duì) SPIF 可成形性影響的發(fā)表期刊論文,因此它不能被納入本文獻(xiàn)綜述。刀具路徑參數(shù) SPIF 中使用的刀具路徑可以等同于 Z 級(jí)加工等加工操作,盡管刀具不切割材料。刀具的運(yùn)動(dòng)由加工操作中使用的相同參數(shù)確定。進(jìn)給速度是刀具在紙張上移動(dòng)時(shí)的速度,通常以毫米/分鐘為單位。逐步減少工具在每個(gè)電路中壓入紙張的距離。主軸轉(zhuǎn)速是指工具旋轉(zhuǎn)的速度,以每分鐘轉(zhuǎn)數(shù)(rpm )表示。刀具路徑參數(shù)通過刀具的運(yùn)動(dòng)和旋轉(zhuǎn)來影響產(chǎn)生的摩擦力[18],進(jìn)給速率和降低速度決定了材料的變形速率。刀具和刀具路徑的相對(duì)旋轉(zhuǎn)方向決定了'銑削模式';無論是傳統(tǒng)還是爬坡銑削,都要使用標(biāo)準(zhǔn)加工條件。如果刀具和刀具路徑都是順時(shí)針或逆時(shí)針移動(dòng),那么是傳統(tǒng)銑削,如果他們正在以不同方向旋轉(zhuǎn),那就是爬銑。攀爬銑削是SPIF 中最常用的模式,因?yàn)楣ぞ咴谛纬蓵r(shí)有效地“滾動(dòng)”在工作臺(tái)上,從而減少了摩擦[19]。雖然切削刀具通常以順時(shí)針方向旋轉(zhuǎn),因此指定刀具路徑方向可能足以讓讀者假設(shè)正在使用哪種銑削模式,但并不像定義常規(guī)或爬升('滾動(dòng)')銑削那樣徹底,因?yàn)樗玫募庸つJ?。幾何圖形增量成形零件的形狀影響應(yīng)變,從而影響材料的成形性。由于分析研究論文中使用的許多不同形狀和尺寸的復(fù)雜性,幾何學(xué)是本文獻(xiàn)綜述中未涉及的一個(gè)方面。在制造實(shí)用部件時(shí),可以在部件的 CAD 模型上執(zhí)行拔模角度分析,以突出顯示哪些壁面比特定角度更陡。在分析中使用該材料的最大壁角將顯示是否有任何部分可能太陡,無法在單個(gè) SPIF 通道中形成。如果沒有比允許的角度更陡峭的部分,則部件很可能會(huì)成功[20]。部件的曲率從直壁到緊密曲線影響過程中形成的應(yīng)變類型(平面,雙軸) 。例如 Filice 等人所介紹的圓錐截頭圓錐體的應(yīng)變,其半徑為 100 毫米,而圓錐體的圓錐體的邊緣為 10 毫米半徑。實(shí)驗(yàn)參數(shù)由于該過程是第一次開發(fā)的,因此對(duì)基本的 SPIF 過程進(jìn)行了修改研究。例如,已經(jīng)探索了許多不同的加熱工件的方法。杜弗歐等人。 [23]在2007 年使用激光將 TiAl6V4 片材的 Fmax 提高了 208 以上。同樣的鈦合金被加熱到 400℃,帶式加熱器安裝在坯料上持有人 Palumbo 和 Brandizzi [24],并觀察到成形性的改善。通過刀具和片材的電流的使用近年來已經(jīng)被探索,也被應(yīng)用于 TiAl6V4 [25]和其他材料,如 AA6061-T6 [26],從而提高了成形性。由于這篇文獻(xiàn)綜述研究了基本 SPIF 的工藝參數(shù),與熱 SPIF 和電 SPIF 相關(guān)的參數(shù)未被檢查。這項(xiàng)研究的問題文獻(xiàn)中關(guān)于 SPIF 工藝參數(shù)對(duì)成形性影響的說法是什么?假設(shè)每個(gè)參數(shù)都有一個(gè)最佳的工作范圍,它是其他參數(shù)的函數(shù)。它們以各種組合相互依存。方法這篇文獻(xiàn)綜述是作為“系統(tǒng)的定量文獻(xiàn)綜述”進(jìn)行的。這種類型的文獻(xiàn)綜述被定義在 Pickering 和 Byrne [27]中。該過程已經(jīng)過多名學(xué)生和研究人員的測(cè)試,并產(chǎn)生可重復(fù)和高質(zhì)量的結(jié)果。由于數(shù)據(jù)輸入的定量特性,該技術(shù)非常適用于 SPIF 參數(shù)區(qū)域,并且可以在列表結(jié)果中看到,可以有效比較多篇論文之間的參數(shù)值。本評(píng)價(jià)中所選研究的參數(shù)對(duì)于每次單程 SPIF 過程都是通用的,并且更多專門的過程(如應(yīng)用電流或外部熱量)未得到解決。許多 SPIF 論文已經(jīng)發(fā)表在可成形性領(lǐng)域,但是下面強(qiáng)調(diào)的納入標(biāo)準(zhǔn)被用來選擇高質(zhì)量的論文集,這些論文可以很好地表達(dá)更廣泛的領(lǐng)域。